We show strong electron correlation has severe effects to conical intersection topographies in minimum energy conical intersections of DNA/RNA nucleobases
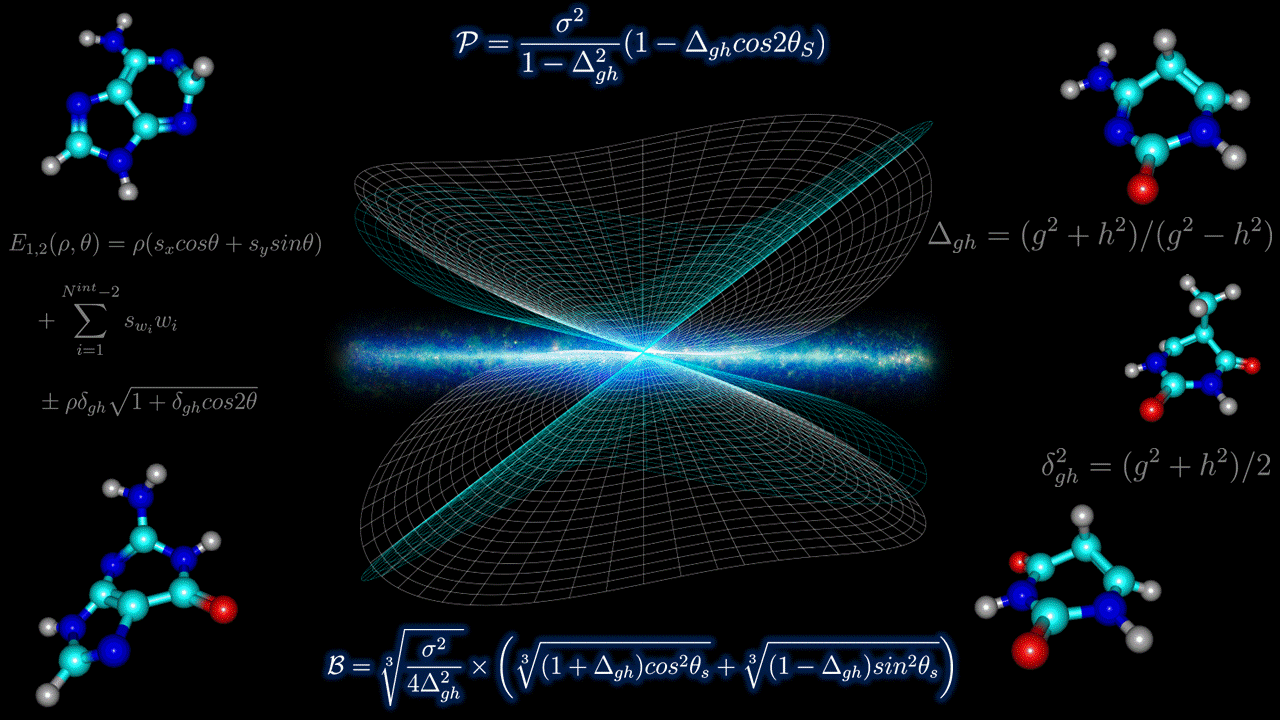
Electron correlation is a key ingredient for adequately describing photo-processes and understanding photo-reactivity from a theoretical standpoint. It is known to affect electronic states differently,[1] and a balanced description is therefore paramount to adequately model non-adiabatic photo-processes involving multiple electronic states.
DNA/RNA nucleobases are widely studied in the literature due to their outstanding photophysical properties. Upon radiation absorption, these systems are able to dissipate the excess energy gained at ultrafast timescales, a feature that has been linked with their photo-protective design nurtured through evolution.[2] Such ultrafast events are mediated by accessible conical intersections, which mediate ultrafast population transfers leading to the ground state and that underpin the photostability of our genetic material.
This work, in collaboration with Roland and Ignacio at Uppsala, Marco and Ivan at Bologna, and Francesco at Laussane, thoroughly analyses for the first time how strong electron correlation affects conical intersection topography in DNA/RNA nucleobases.[3] We do this by employing a systematic procedure where we start from spaces including all π and π*, as well as n lone pair valence orbitals and reduce them one by one by reremoving the least contributing active space orbital according to their occupation number. We consider most photochemically relevant conical intersections on all 5 systems: uracil, thymine, cytosine, adenine and guanine. This led to >300 different optimised minimum energy conical intersections, considered over two separate one-electron basis set contractions, which can be found in an open repository.[4]
Our simulations show how conical intersection topography depends very strongly on active space size, this being particularly relevant for the well-known "ethylene-like" (ring-puckered) conical intersections. Despite featuring vastly different topographies, we find the geometries to be very similar across the different active spaces, thus ruling out a geometric effect and emphasising the importance of appropriately describing the partaking electronic states in the crossing.
Comparison across systems shows different topographies for the "ethylene-like" intersection: this could conflict with the hypothesis all nucleobases share a unified decay mechanism,[5] which is responsible for their shared photo-protection properties.[6] On the other hand, the different ππ*/nπ* crossings responsible for population transfer to dark nπ* states show, for the most part, a shared topography across species.
This study highlights the importance of strong correlation at crossing regions, and cautions against using small active spaces for determining conical intersection topographies, as these show the largest deviations in character.
References
[1] J. Segarra-Martí, M. Garavelli and F. Aquilante, "Multiconfigurational Second-Order Perturbation Theory with Frozen Natural Orbitals Extended to the Treatment of Photochemical Problems", J. Chem. Theory Comput. 2015, 11, 3772-3784.
[2] S. Boldissar and M. S. de Vries, "How nature covers its bases", Phys. Chem. Chem. Phys. 2018, 20, 9701-9716.
[3] J. Cuéllar-Zuquin, A. J. Pepino, I. Fdez Galván, I. Rivalta, F. Aquilante, M. Garavelli, R. Lindh and J. Segarra-Martí, "Characterising conical intersections in DNA/RNA nucleobases with multiconfigurational wave functions of varying active space size", J. Chem. Theory Comput. 2023, DOI: 10.1021/acs.jctc.3c00577. Preprint available here: https://doi.org/10.26434/chemrxiv-2023-m5s0w
[4] J. Cuéllar-Zuquin, A. J. Pepino, I. Fdez Galván, I. Rivalta, F. Aquilante, M. Garavelli, R. Lindh and J. Segarra-Martí, https://doi.org/10.5281/zenodo.8348402
[5] M. Merchán, R. González-Luque, T. Climent, L. Serrano-Andrés, E. Rodríguez, M. Reguero and D. Peláez, "Unified Model for the Ultrafast Decay of Pyrimidine Nucleobases", J. Phys. Chem. B 2006, 110, 26471–26476.
[6] R. Borrego-Varillas, A. Nenov, P. Kabaciński, I. Conti, L. Ganzer, A. Oriana, V. K. Jaiswal, I. Delfino, O. Weingart, C. Manzoni, I. Rivalta, M. Garavelli and G. Cerullo, "Tracking excited state decay mechanisms of pyrimidine nucleosides in real time", Nat. Commun. 2021, 12, 7285.